Unraveling the Mysteries of Electroencephalography: Dive into How it Works
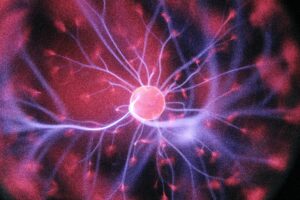
The human brain, with its billions of interconnected neurons, is a marvel of complexity.
It governs our thoughts, emotions, and actions, yet much of its inner workings remain shrouded in mystery.
Electroencephalography, a groundbreaking technology, allows us to peer into the depths of the mind and decipher its language.
In this article by Wellix, we will take you on an in-depth journey into the fascinating world of encephalography, exploring its mechanisms, techniques, and the science behind this remarkable tool.
The Basics of Electroencephalography
At its core, electroencephalography is a non-invasive method used to measure and record the electrical activity in the brain.
It relies on the fact that when neurons communicate with each other, they generate electrical impulses, creating intricate patterns of brain waves.
These waves serve as a window into the mind, reflecting different mental states and processes.
Electrodes and Brain Wave Detection
To capture these elusive brain waves, electroencephalography employs a device called an electroencephalograph (EEG).
The EEG is a non-invasive device that plays a vital role in capturing the electrical activity of the brain and translating it into actionable data.
Capturing the Brain’s Electrical Symphony
The EEG works by detecting and recording the electrical signals generated by the neurons in the brain.
It consists of multiple electrodes that are strategically placed on the scalp to detect the electrical impulses produced by the brain’s neural network.
These electrodes act as sensors, picking up tiny electrical fluctuations and translating them into measurable signals.
Electrodes and Sensor Placement
The electrodes used in EEG are typically small metal discs or sensors that are attached to the scalp using a conductive gel or paste.
The placement of these electrodes follows specific international standards, such as the International 10-20 system, which ensures consistency and accuracy in data collection across different individuals and research settings.
By positioning the electrodes at different locations on the scalp, the EEG can capture electrical signals from various regions of the brain.
Amplification and Signal Processing
Once the electrical signals are detected by the electrodes, they are amplified to make them easier to measure and analyze.
The amplified signals pass to a recording device, which converts them into a visual representation.
The EEG consists of a series of waveforms that reflect the brain’s electrical activity.
Advancements in EEG Technology
Over the years, advancements in EEG technology improved significantly its capabilities.
Modern EEG devices are more portable, user-friendly, and capable of collecting data with higher precision and resolution.
Researchers also explore wearable and wireless EEG systems that offer greater freedom of movement and allow for continuous monitoring in real-world environments.
Different Types of Brain Waves
The brain wave patterns recorded by electroencephalography can be categorized into several types, each associated with different mental states and activities:
- Delta Waves (0.5-4 Hz): Delta waves are observed during deep sleep or in certain pathological conditions. They are slow in frequency and high amplitude.
- Theta Waves (5-8 Hz): You can see Theta waves during states of relaxation, daydreaming, and light sleep. They are also associated with creativity and access to the subconscious mind.
- Alpha Waves (9-12 Hz): Alpha waves occur when the mind is in a relaxed but alert state, such as during meditation or when closing one’s eyes and taking a break from visual stimuli.
- Beta Waves (13-30 Hz): Beta waves are associated with active thinking, problem-solving, and focused concentration. They last during periods of heightened mental activity and engagement.
- Gamma Waves (31-100 Hz): Gamma waves are the fastest brain waves and are linked to advanced cognitive processes, including memory formation, attention, and perception.
Analyzing Brain Wave Patterns
Electroencephalography’s true power lies in the analysis of these brain wave patterns.
Experts, such as neuroscientists and clinicians, carefully examine EEG recordings to extract valuable information about an individual’s mental state and processes.
Brain Wave Synchronization and Desynchronization
One crucial aspect of analyzing brain waves is the synchronization and desynchronization of different frequency bands.
For instance, increased synchronization of alpha waves often indicates a state of calm and relaxation, while desynchronization of beta waves suggests heightened mental activity.
Event-Related Potentials
Electroencephalography also detect event-related potentials (ERPs), which changes in the brain wave patterns that occur in response to specific stimuli or events.
ERPs provide insights into cognitive processes such as attention, memory, and sensory perception.
Signal Processing and Advanced Analysis Techniques
To make sense of the vast amount of data generated by electroencephalography, they employ signal processing, and advanced analysis techniques.
These techniques involve filtering the EEG recordings, isolating specific frequency bands, and applying statistical algorithms to extract meaningful information.
Some techniques and signal processes are:
Filtering:
Filtering is a fundamental step in electroencephalography signal processing.
It involves removing noise and unwanted artifacts while preserving the essential brain wave signals.
Common filtering techniques include high-pass filters, low-pass filters, and band-pass filters.
High-pass filters remove slow-frequency components, such as those associated with movement or electrode artifacts.
Low-pass filters eliminate high-frequency noise, while band-pass filters isolate specific frequency ranges of interest, allowing researchers to focus on particular brain wave bands.
Time-Frequency Analysis:
Brain wave patterns are not static but vary over time.
Time-frequency analysis techniques enable researchers to examine how brain wave power and frequency change dynamically during different tasks or stimuli.
One popular method is the Short-Time Fourier Transform (STFT), which reveals how the power spectrum of brain waves evolves over time.
More advanced techniques, such as wavelet transforms and spectrograms, offer enhanced time-frequency resolution, enabling the detection of transient changes in brain activity.
Event-Related Potentials (ERPs):
Event-Related Potentials (ERPs) brain wave responses occur in relation to specific events or stimuli.
Analyzing ERPs provides insights into cognitive processes and neural responses to external stimuli.
Techniques such as averaging and epoching isolate and extract ERPs from the electroencephalography data.
Statistical analysis methods, such as scalp topography and cluster-based permutation tests, help identify significant ERP components and differentiate brain responses between experimental conditions.
Spectral Analysis:
Spectral analysis examines the frequency composition of brain wave signals.
By decomposing the electroencephalography data into different frequency bands, researchers gain insights into the relative power and coherence of brain waves at different frequencies.
Methods like the Fast Fourier Transform (FFT) or the more advanced multitaper spectral analysis provide information about the dominant frequency components, allowing for the identification of specific brain wave bands and their relationship to cognitive processes.
Connectivity Analysis
Connectivity analysis explores the functional connections and interactions between different brain regions.
It examines how brain waves from different regions synchronize or correlate with each other.
Measures such as coherence, phase-locking, and Granger causality analysis quantify and characterize these inter-regional relationships.
Connectivity analysis helps researchers understand the neural networks involved in specific tasks or cognitive functions and provides insights into brain connectivity disruptions in neurological disorders.
Machine Learning
Machine learning techniques are increasingly being applied to electroencephalography data analysis.
These algorithms can identify patterns, classify brain states, and even decode the intentions of individuals using BCIs.
Methods such as support vector machines (SVM), artificial neural networks (ANN), and hidden Markov models (HMM) train models that can predict cognitive states, recognize mental tasks, or translate brain wave patterns into control signals for external devices.
Applications of Electroencephalography
Electroencephalography wide range of applications applies in both research and clinical settings.
It aids in the diagnosis and treatment of neurological disorders, the study of cognitive processes, and the exploration of brain-computer interfaces (BCIs) that allow individuals to control external devices using their thoughts.
The Brain is More Complex and More Mysterious than We Think
Electroencephalography is a remarkable technology that has revolutionized our understanding of the human brain.
By deciphering the intricate language of brain waves, we gain insights into our mental states, cognitive abilities, and emotional well-being.
As electroencephalography continues to advance, its potential to unlock the mysteries of the mind pave the way for groundbreaking discoveries becomes increasingly evident.
References
Information about electroencephalography – PubMed (nih.gov)
Electroencephalography | Definition, Procedure, & Uses | Britannica
Electroencephalography – an overview | ScienceDirect Topics
by Alonso Ruiz